Active Flow Control has long been investigated as an alternate means of flight control for aircraft. While it has recently matured into a fully-fledged control method for a DARPA “X-plane”, it has also been recognized as a way to control lasers.
For several years, the US Air Force Research Laboratory (AFRL) has undertaken virtual, ground and in-flight testing using active flow control (AFC) to neutralize the effects of aerodynamic disturbances on a laser beam director. Employing AFC in conjunction with selected beam director optical components has shown promise in improving the fidelity, concentration and direction of laser energy projected from aircraft at high speeds.
The AFC-influenced beam director concept has been evaluated and flight-tested by the AFRL’s Hybrid Aero-Effect Reducing Design with Realistic Optical Components (HARDROC) team. The team includes researchers from the Lab’s Wright-Patterson Air Force Base (AFB)-based Aerospace Systems Directorate, its Directed Energy Directorate at Kirtland AFB, New Mexico, and prime contractor MZA Associates. MZA is a leading High Energy Laser (HEL) modeling, analysis, design, testing and integration firm based in Albuquerque, New Mexico.
The HARDROC team’s research may enable the practical use of high-energy lasers on fast, dynamically maneuvering aircraft like fighter jets. Until recently, most of the popular discussion of fitting laser weapons to fighter and attack aircraft has focused on power limitations.
But ensuring that the beam weapons they might carry can readily and accurately be aimed at targets is just as daunting a challenge. A large segment of that challenge lies in overcoming aerodynamic turbulence.
A Turbulent Situation
Aiming or steering laser beams and pulses to a target can be thrown off by substances in the atmosphere such as water vapor, sand, dust, salt particles and smoke. These substances absorb and scatter light. But turbulent airflow can also defocus a laser beam.
The airflow around the contours of an airplane changes with speed, angle of attack, and yaw. The changes can be highly localized, affecting portions of an airframe where sensors or fire direction devices like laser beam directors are placed. Turbulent or continually changing airflow across a beam director can cause scintillation, beam wandering and phase front distortion.
HEL researchers and designers have previously sought to counter the interference effects of disturbed airflow by using adaptive optics, which make rapid, fine adjustments to a laser beam continuously in response to observed turbulence.
But rather than attempting to control beam focus and steering by compensating for continual changes in the local environment around a beam director, an alternate strategy is to try to shape that local environment itself using active flow control. In essence, this is the same task to which AFC has been yoked to influence airflow across aerodynamic surfaces for flight control.
AFC relies on timed and metered pulses to change airflow, precisely placed disruptions at a specific location. The devices/systems that actuate flow changes are called “effectors” and are characterized as “blowing”, via a pneumatic or electric system.
For example, one technique puffs or streams air extracted from a jet engine through one-to four-millimeter-wide holes in the relevant parts of an aircraft’s skin. Conversely, air can be sucked out of the flow. Such pneumatic blowing and suction can create or shape aerodynamic wakes.
Effectors including arrays of fluidic oscillators, shaped as slots, trapezoids or other configurations or sweeping jets can inject air into the flow at various locations on an aircraft, influencing the local conditions.
Electrical power can also be used to discharge aerodynamic or electrical pulses to alter airflow locally. Plasma actuators discharge pulses of electricity into the air through electrodes. The heat from the electricity turns the air into plasma, thermally altering flow vectors.
Electrically actuated synthetic jets, millimeter-wide openings in the aircraft surface that open into centimeter-wide cavities with diaphragms rely on rapid voltage pulses to make diaphragms oscillate inward or outward, creating airflow sucking or blowing effects, much like a human lung.
The Beam Director
Information about specific elements of HARDROC, including its beam director, is hard to come by. The AFRL cites operational and security concerns in its replies to questions. Among the most pertinent questions are which AFC methods were considered as part of their testing, and which was found to be the most effective.
An evaluation of the various methods and effectors was undertaken initially using computational fluid dynamic (CFD) simulation techniques, according to Scott Sherer, HARDROC CFD lead.
Sherer, nor anyone else connected with the program, would comment on which effector strategy worked best in simulation and later in ground/flight testing. He did note that, “We were able to demonstrate significant reduction in aero-effects across a range of speeds and look angles.”
MZA Associates was tasked with designing and developing the low-power, sub-scale beam director that was used. The director was tested both in a scaled wind tunnel environment and on a business jet in flight. As far back as 2007, MZA participated in AFRL-sponsored wind tunnel testing of adaptive optics in combined aero optics and free stream turbulence experiments.
The company’s previous work in adaptive optics, a system called WaveTrain, atmospheric characterization devices and HEL metrology tools suggest which AFC techniques are most likely to be effective in turbulence mitigation for laser beams. So could the test settings, which included an environmental chamber in addition to the wind tunnel and airborne tests.
Flight testing saw the beam director affixed to a Dassault Falcon 10 jet, owned by an air parts business in Oklahoma City, USA. The Falcon 10 can cruise at around 430mph (700km/h), but its twin engines produce only 7,000 lbs of thrust, which isn’t substantial in a 20,000 lbs maximum gross weight business jet.
The thrust available for pneumatic blowing and suction techniques like those described previously would be limited. The engine placement would also be less than favorable for piping bleed-air to a beam director located on the port side of the fuselage aft of the flight deck.
Likewise, the AFRL featured a photograph of the aircraft in a press release about HARDROC, but it was edited to remove various details. Other photos of the aircraft show little in the way of visible effectors on the aircraft skin around the beam director.
These details and the fact that the beam director was illuminated by a low-power scoring laser fitted to a second Falcon 10 flying in formation during an experimentation flight could suggest that the HARDROC team found an electric AFC effector strategy using plasma actuators or pulsed synthetic jets was found to be the most effective – at least with the subscale beam director flown.
Rudy Johnson, HARDROC program manager said, “The biggest question in our mind was if these flow-control techniques could be used with the sensitive optical components required for an advanced directed energy system. HARDROC answered that question with an emphatic yes.”
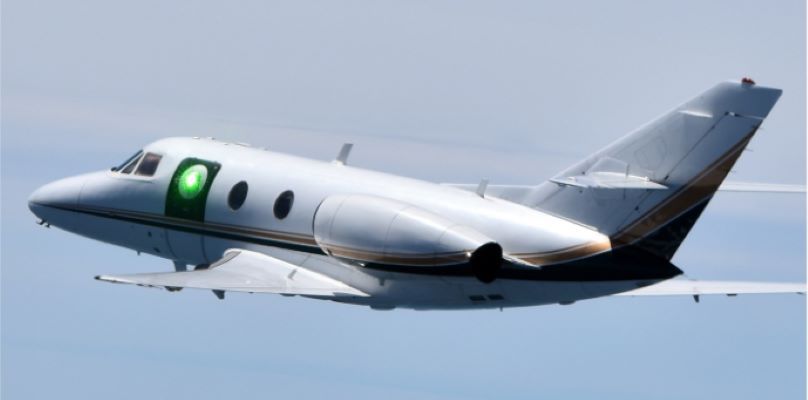
Johnson’s framing of the question regarding flow control techniques might suggest concern about the mechanical impact of blowing actuators on optical components. But given the digitally-controlled configuration of the beam director on the test aircraft, the framing may very well may point to concern about electromagnetic interference.
The flat-panel beam director looks as if it could be a phased array system. Such systems can be extremely compact. A full 50cm (20in) diameter beamlet array steerable over a 100° cone may take up a volume of just 0.037m3.
The Falcon 10 cruised at high speed during flight tests in August 2022 and a variety of sensors were used to measure aerodynamic disturbances according to the AFRL. The HARDROC beam director reportedly enlarges the envelope that airborne directed energy systems can operate in, providing 360° field of regard across extended speed regimes with reduced size, weight and power, or SWaP, compared to other state-of-the-art turrets.
This advance would indicate that HARDROC is on a path to integrating the new beam director onto fast tactical aircraft. In an AFRL release, Mike Stanek, technical advisor for the Aerospace Systems Directorate’s Integrated Systems Branch, says as much:
“The successful flight demonstration of the HARDROC turret clears one of the key remaining technological hurdles for the operation of high-power lasers on high-speed aircraft for a variety of Air Force missions. Integration of the low-SWaP HARDROC turret would allow less laser power to be lost to aero-effects, thus enabling mission performance compared to other types of integration strategies.”
What Stanek and others from the HARDROC team have not said is that AFC may not just be useful as a flight control or laser focusing method – it may find application in conjunction with other vehicle sensors whether in the air or under the water.