by Abigail Williams
On average, commercial aircraft are struck by lightning approximately once per year –potentially causing extensive damage or even in extreme cases, the loss of an aircraft. In recognition of this risk, lightning strike protection is a vital element of aircraft design.
So, how does lightning strike protection on aircraft work? What are the key operational steps and standards requirements that must be met in aerospace for lightning tests? How are engineers meeting the challenge of lightning striking composites through technology and testing? And how best can lightning testing and standards continue to meet the needs of changing aircraft designs and advances in materials?
Types of effect
Commercial aircraft are struck by lightning approximately once every 3,000 flight hours, equating to around once per year, and strikes could potentially lead to the loss of an aircraft if it is not properly designed. In recognition of this ongoing risk, aircraft are required by the FAA to be designed and maintained to survive the direct and indirect effects of lightning strikes.
As Dan Morgan, laboratory manager and technical advisor at Element Oxford, part of Element Materials Technology Warwick in the UK explains, direct effects are the result of the lightning arc physically attaching the to the aircraft and indirect effects are the result of the current spreading out through the aircraft. These effects are further split into additional categories that impact fuels, structures and avionics, as well as into protection required for aluminum aircraft and composite aircraft.
Terry McVenes is president and CEO of the US-based Radio Technical Commission for Aeronautics (RTCA), which develops technical guidance such as the Environmental Test standard DO-160, widely used by government regulatory authorities and industry. He says that because lightning cannot be prevented from striking an aircraft, designers “must use methods for that energy to pass through the aircraft without too much of it going to the wrong places and causing damage and upset”.
Once designed, extensive testing is then carried out to “make sure that the designer got it right”.
“Composites are more challenging to protect than aluminum, which is one of the best conductors in the world. This makes lightning design easier – not necessarily easy, just easier than composites. Still, designers have done a tremendous amount of research, development and testing to make composite aircraft as safe as aluminum during a lightning strike,” says McVenes.
“As a former airline pilot, I’ve taken multiple lightning strikes during my career. I know first-hand about the importance of protecting an aircraft from the results of a lightning strike in maintaining safe and reliable operations of the aircraft’s components and systems,” he adds.
Direct effects
Approaches to the design of lightning protection systems for aircraft structures generally differ depending on whether aluminum or Carbon Fiber Reinforced Polymer (CFRP) composites are used. For aluminum structures, as long as a sufficient thickness of metal is used, Morgan says it will be puncture free and that for large parts of the aircraft that aren’t in initial attachment areas, 2mm thick aluminum is sufficient.
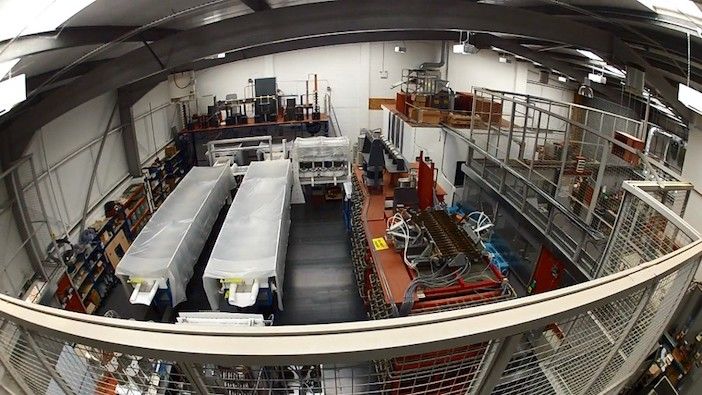
is possible
On the other hand, composite structures will normally require a layer of metallization on top of the CFRP, typically a copper or aluminum mesh, though metallic paint sprays are also used. This is complicated by the fact the paint on top of the metallization affects its performance, so it can be challenging to protect CFRP that also has thick layers of paint.
“Structure doesn’t necessarily need to be damage or puncture free as a result of lightning strikes, so often a balance is required between the cost and weight penalty protection requires, and the amount of damage that can be tolerated,” says Morgan.
“Normally small panels of around 500x500mm are tested rather than full structures, especially at early stages of the design. This allows a number of different protection methodologies and structures to be tested. Full structures may then be tested at the certification stage, though small panels are still often used,” he adds.
For fuel systems, Morgan says that lightning protection and testing generally follows similar lines to those used for structures, “except that the fuel tank cannot be punctured so needs to be fully protected”.
For avionics, although the majority of such systems are inside the aircraft, Morgan observes that any that could be exposed to a direct lightning strike “are unlikely to be able to withstand it so are often protected by a lightweight fairing, which is often there for aerodynamic reasons as well”.
“Fairings are often tested in a similar way to structure, though sometimes puncture is acceptable, but only if the high current arc doesn’t attach to the underlying avionics – in which case, a full-scale test is performed with the fairing and simulated structure underneath. Any arc attachment to the underlying structure results in current flow, which can be monitored. This test can also be performed as a panel test,” he says.
Indirect effects
According to Morgan, protecting structures from indirect effects is important because control surfaces have to survive not only a direct attachment, but the hinges, bearings and actuators will also need to conduct high levels of current that will transfer from the control surface to the rest of the aircraft without being damaged. This often means providing multiple conductive paths, so that the current can be divided and the amount of current each part and component takes is reduced.
“Testing normally requires the full structure so that current can distribute representatively. However, if this isn’t possible, single actuators and hinges can be tested on their own using worst case assumptions to determine the test level,” says Morgan.
According to Morgan, fuel systems are the largest topic of indirect effect protection because the internal structure of a wing is very complex with a large number of bolted interfaces, both between the skin and internal structure and between the internal components.
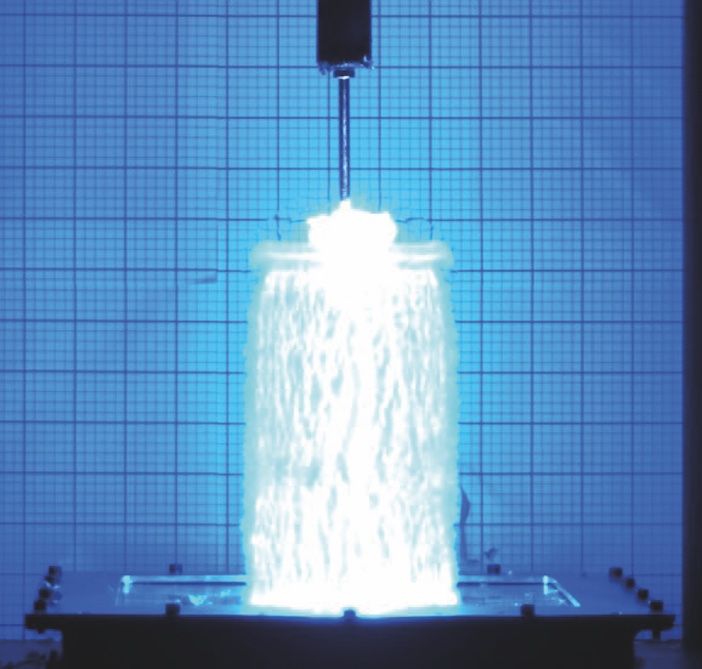
“All of the structure will carry current in the event of a lightning strike to a wing tip, as the current will need to travel to the exit location on the aircraft. None of the interfaces can spark as current travels through them, as this would be an ignition risk,” he says.
“For Part 25 aircraft, multiple layers of protection are used and, when tested, several of the layers are purposefully disabled so that the design can be shown to be safe even in combination with faults and failures. Some of the protection includes counter sunk fasteners, sealant, interference fit fasteners, and coatings to improve conductivity. Protection is particularly complex with CFRP,” he adds.
At Element Oxford, fuel tank tests are performed either in a dark enclosure with a camera looking at the interface to see if any sparking or outgassing occurs. Tanks tests are also conducted in an enclosure that is filled with a diagnostic gas replicating fuel vapour, which will detonate in the event of sparking or outgassing.
“Fuel tank tests are only very rarely carried out on large scale parts due to the complexity of manufacturing the part, as well as performing the test, because it’s very difficult to determine what caused an ignition hazard,” says Morgan. “Tests are almost always carried out on coupons replicating the interfaces.”
In a similar way to fuel systems, Morgan observes that avionics have to deal with current being distributed through the airframe, as well as the induced effects of voltage and current, due to the penetration of magnetic fields through the fuselage of the aircraft, which is greater for CFRP than aluminum.
Cable routing is also very important to minimize induced effects, as well as to isolate sensors and provide surge arrestors with GDTs [Gas Discharge Tubes] and transorbs at the avionics inputs.
In terms of avionics testing for indirect effects, Morgan explains that different waveforms replicating the threat in an aircraft are injected into the avionics system to see if it causes damage or upsets/failures. This is mostly a bench top test performed on the avionics system – however, for critical systems, Morgan says it “has to be proven to at least the level they will see in the event of a lightning strike.”
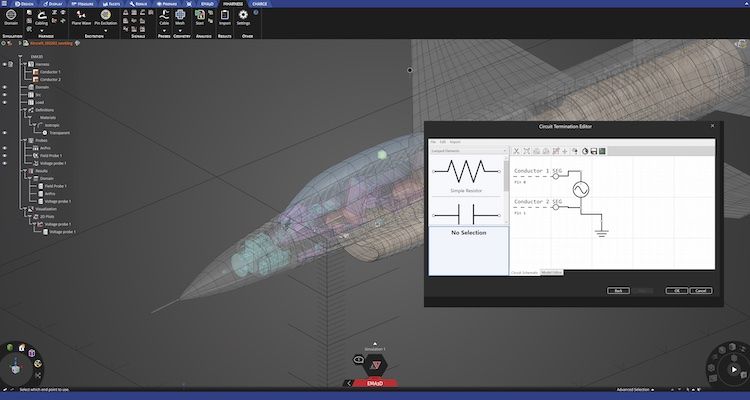
“This requires a whole aircraft test, where low levels of lightning are injected into a full aircraft, and the resulting currents and voltages are physically measured at the inputs of the critical avionic systems. The results are then extrapolated to a full threat lightning strike, a margin is then added, and these are the levels the critical systems are tested to,” he says.
“A whole aircraft isn’t available until the end of the certification, but the avionics need to be tested many months or even years in advance, so testers have to estimate or model the test levels, which are then confirmed during a whole aircraft test,” he adds.
Evolving standards
When it comes to ongoing advances in lightning protection, Morgan observes that the fundamentals haven’t changed significantly – but confirms that, as a lab, Element has improved its diagnostics and automation processes to improve efficiencies in testing.
“In terms of lightning protection technology, there are several companies working to eliminate the need for external metallisation by improving the resistivity of the carbon, so it is less damaged during a lightning strike. Typically, this is involves doping the epoxy with carbon nanotubes, or graphene, or metal particles,” he says.
Looking ahead, Morgan also explains how he and his colleagues at Element are supporting the EUROCAE standards committee as it works to develop standards, particularly for electric propulsion and vertical takeoff and landing aircraft.
“These documents are in the early stages, but are likely to be predominantly based on existing standards and no new tests are expected – as the lightning strike, and how it interacts with the aircraft itself isn’t changing,” he adds.
Meanwhile in the USA, McVenes observes that each time a new material, new aircraft design, or new use for an aircraft arises the industry – including organizations like the FAA and RTCA – must “re-evaluate all the data, steps, and assumptions” relating to lightning protection systems and testing. If any of these variables have changed, the resulting environments and test methods must then be changed to compensate.
“For example, with new eVTOL aircraft, the use of the aircraft is significantly different than a conventional aircraft, and so are some of the systems. Therefore, does that change the environment or exposure? Do the new materials or systems tolerate lightning as well as the previous materials?
“If not, design and testing must change to guarantee that the resulting aircraft is just as safe as earlier designs,” he adds.
Protecting rocket launches from strikes
An intriguing aspect of lightning protection is the variety of approaches adopted to protect spacecraft at rocket launch pad sites.
Carlos Mata, chief technology officer at Scientific Lightning Solutions, which designed the LC-39B lightning protection system (LPS) at Kennedy Space Center (KSC) explains that one of the reasons Lightning Protection Systems (LPS) are deployed to protect spacecraft is because spacecraft made of composites “do not have lightning protection, meaning we cannot afford for lightning to attach to them.”
According to Mata, the KSC system is a conventional, isolated LPS that uses catenary wire systems to intercept lightning thus “minimizing the probability of direct attachment to the flight hardware and ground support equipment”.
Another aspect of the LPS that Mata observes most people may not be aware of is a “comprehensive bonding, shielding, and grounding scheme, which minimizes the lightning-induced transients on the flight hardware and ground support equipment”.
“The system was designed with a software-based Monte Carlo tool we developed in house, which allows us to estimate the probability of lightning attachment to structures and fine tune the design to achieve optimum performance,” he says.
“On average, LC-39B has been subjected to about 30 strikes per year since the LPS was installed in 2011, and no strike has caused any problems at the pad, including one very large positive that terminated on the LPS with the vehicle at the pad,” he adds.
For Mata, the main difference between a launch pad and an aircraft LPS is that the launch pad LPS is isolated. In other words, there are no lightning currents flowing near the structure or spacecraft being protected, whereas the aircraft’s lightning protection system is “something that the aircraft has to carry with it, for instance, metal mesh on the outside of the composite”.
“There is one challenge. That is that laboratory test equipment does not quite reproduce the lightning environment, which in some applications is OK, but in some others – for instance wind turbines – it is not,” he says.
Simulating lightning strikes gets sophisticated
The effective and accurate simulation of lightning strikes forms an important part of lightning testing. As Tim McDonald, president at Electro Magnetic Applications explains, the first step in designing lightning simulation systems for aerospace applications is to determine where lightning will attach on an aircraft.
“This location of attachment is a critical issue for aircraft design. More than 90% of aircraft lightning strikes are triggered by the presence of the aircraft itself,” he says.
Next, engineers must determine how the current from lightning will flow on electronics systems and their cables. Testing and system design in response to these indirect effects of lightning involves the engineering of cable harnesses, as well as specially designed circuits to withstand the voltages produced by a lightning strike. Finally, McDonald points out that design teams must test and design systems to deal with the physical damage of lightning – or direct effects. “In such cases, structures may be damaged beyond the point of being able to provide their original function. Or it might cause an ignition of fuel vapors within the aircraft fuel tanks,” he says.
“Aircraft certification for lightning effects involves a combination of laboratory testing and three dimensional electromagnetic simulation. Most physical testing involves coupons or sub-structures that must be evaluated for damage. Further, the electronics equipment is tested alone with the lightning levels determined from simulation. Full aircraft testing is typically focused on validating simulation models and increasing their accuracy,” he adds.
More broadly speaking, McDonald observes that lightning simulation “has matured to a high degree of fidelity and accuracy” and has been used successfully for over three decades. “Many of the techniques used today were pioneered on aircraft programs long ago. However, they are increasingly sophisticated and handle a greater degree of complexity. There is a focus in using computer-aided design files that describe the aircraft to ensure that the simulation corresponds to the actual drawings used to build the aircraft,” he says.
Moving forward, McDonald is confident that evolving lightning simulation systems will continue to meet the needs of changing aircraft designs and new materials, while simulation will be a vital part of lightning design and certification.
“Simulation’s role is only increasing as time passes. There will always be a role for both simulation and testing. By doing both testing and simulation at a high level and correlating the results of each in an organized way, engineers actually learn more about how lightning interacts with aircraft than if they were limited to either simulation or testing.”