Words by Eric Tegler
As 2021 drew to a close, reports that China would soon unveil an advanced hypersonic wind tunnel capable of simulating objects flying at Mach 30 sparked both alarm in the West and assertions from experts that no such tunnel is really on the horizon.
Even if China’s rumored 15GW-powered JF-22 tunnel is real, it simply cannot cover the spectrum of development needed in the race to field hypersonic weapons and hypersonic aircraft. As Purdue University professor of aeronautics and astronautics, Joseph Jewell says, “No one facility on the ground can reproduce all aspects of hypersonic atmospheric flight.”
Purdue University has a long-standing hypersonics research department and a new Hypersonics and Applied Research Facility (HARF) under construction at its West Lafayette, Indiana campus. They are emblematic of a push, particularly in the USA, to deter extant and emerging Chinese and Russian hypersonic weapons by counter-developing and fielding hundreds of hypersonic weapons of different types by the mid 2020s.
Back in April, Mark Lewis, director of research and engineering for modernization at the US Department of Defense, confirmed hypersonics as one of the Pentagon’s top priorities. Development plans cover Mach 10 rocket-powered boost-glide missiles to Mach 5 air-breathing cruise missiles on the table.
Given the urgency of hypersonic research, there is a demand for test and development facilities, particularly advanced hypersonic wind tunnels. They are key to understanding how to configure projectiles to withstand the unprecedented temperatures and air flow surrounding hypersonic vehicles during flight.
The threshold for what is considered hypersonic speed is generally agreed to be around Mach 5 and large hypersonic wind tunnels are rare. Most existing facilities in the USA were designed and built from the 1950s to the 1970s, when America invested heavily in missile development and the Space Program. The tunnels can produce hypersonic flows that are cold, high disturbance, small and relatively short.
While these tunnels are good for basic research and a compartmentalized approach solving hypersonic flight problems, they cannot simultaneously produce hot, low disturbance and lengthy flows at scale. Experts say nothing can outside of actual flight testing.
But there’s a small and growing group of US facilities that can produce the above qualities individually or severally. These advanced hypersonic wind tunnels and their more traditional predecessors are critical parts of national infrastructure in the USA and Europe.
Wind tunnel superpowers
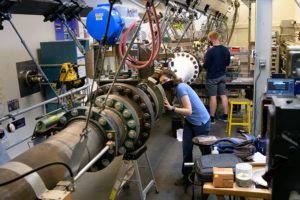
There are five common types of hypersonic wind tunnels. Quiet tunnels are perhaps the most sought after type. Quiet tunnels are so-called because they are capable of flowing air at hypersonic velocities without the turbulence created by the boundary layer that develops at such speeds.
NASA’s Langley Research Center developed the first quiet wind tunnels for supersonic and hypersonic research in the 1980s. They address one of the major challenges in hypersonic flow research – the accurate prediction of transition.
Transition is where smooth or laminar airflow across a surface becomes disturbed or turbulent as airflow velocity increases. Such transition generates significant increase in viscous drag and heat flux, leading to severe restrictions on the performance and thermal protection of hypersonic vehicles.
Purdue constructed one of the first non-NASA quiet tunnels, the Boeing / AFOSR Mach-6 Quiet Tunnel, in the late 1990s. The tunnel is based on a Ludwieg Tube design – a long cylindrical tube downstream from a large air tank and convergent-divergent nozzle controlled by a diaphragm or valve – first developed in the 1950s.
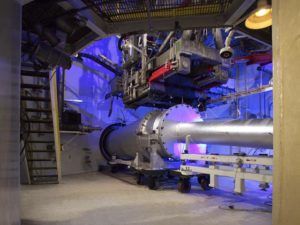
A quiet tunnel siphons off or suctions turbulent air through a bleed slot on the tunnel. This produces smoother, quieter airflow downstream where it passes over a test article like an inlet, wing or fuselage.
Non-quiet tunnels like the Mach 6-plus HTFD hypersonic wind tunnel at Delft University of Technology in the Netherlands, which is also a Ludwieg Tube design produce 2% or higher airflow disturbance from their flow nozzles. This effectively means that the test articles within virtually fly through an air stream with unwanted noise, making it difficult to isolate various measurements from the background.
“Our superpower,” Jewell says, “is that via very careful design of the tunnel and decades of work, we can produce hypersonic flows that have about .02% stream disturbance, basically 100-times smaller. It’s much more representative of the conditions that a real flight vehicle sees in the atmosphere.”
Purdue’s Mach 6 tunnel is still the largest quiet hypersonic wind tunnel in the world with a moderately long flow time of about two seconds. Longer flow time allows engineers greater opportunity to measure and observe. Jewell points out that “if the airflow is traveling at 1000 meters per second, in two seconds a test object has flown two kilometers in the wind tunnel”.
The quiet tunnel has been consistently used for basic physics investigation and hypersonic modeling development with test subjects including cones and varied geometry articles, often representative of missile shapes, placed inside its two foot (0.60m) diameter test section. More recently, objects with notional control surfaces such as wedges have been examined.
Jewell says Purdue researchers have been assessing fixtures like reaction control jets, able to point a vehicle directionally. Two current Air Force Research Laboratory-funded projects are looking into airflow instability which forms on intake lips.
The Mach 6 quiet tunnel will soon be joined by two others in the new US$41 million, 65,000ft2 HARF facility at Purdue. It will house the only Mach 8 hypersonic quiet tunnel facility in the world, which is also a Ludwieg Tube configuration, as well as an advanced materials and manufacturing sensor called HAMTC (Hypersonics Advanced Manufacturing Test Capability).
HARF will also host one of the other major types of hypersonic tunnels, a hypersonic pulse shock tunnel called HYPULSE.
Different tools for different jobs
Shock tunnels, sometimes called blast expansion tunnels, use valves or burst diaphragms with a fast-action release to send hypersonic shockwaves down the tunnel, allowing for the study of high Mach number flows on test articles for around 0.5 to 5.0 milliseconds.
“Behind that shockwave are the conditions you’re looking for,” says Karen Berger, a hypersonic wind tunnel facility manager at NASA Langley in Virginia, USA.
NASA’s Langley Research Facility is home to numerous subsonic, transonic, supersonic and hypersonic tunnels. Berger manages three blowdown hypersonic wind tunnels at Langley. Capable of flowing air at Mach 6 and Mach 10, they were built in the 1960s. Blowdown tunnels are the most common hypersonic tunnel design.
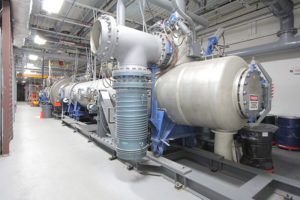
HYPULSE and other shock tunnels like the large CUBRC Aerosciences Group shock tunnel in Buffalo New York, the US Air Force’s Arnold Engineering Development Complex shock tunnel in White Oak, Maryland, the UK’s T6 Stalker Tunnel (T6) at the University of Oxford Osney Mead Estate and the upgraded X3R tunnel at the University of Queensland in Australia, share a basic design layout with the blowdown tunnel.
In a blowdown tunnel the flow process starts with air fed through a dryer to eliminate water molecules. From the dryer it goes into a high pressure chamber where pressure builds as it is injected via a pump. Once a target pressure is reached a valve is opened to allow the air into a plenum which is also regulated at high pressure. The air then passes through a converging-diverging nozzle which narrows at entry and widens at exit. This accelerates the high pressure air to hypersonic speed.
The hypersonic flow travels a few feet further as the tunnel widens to a test section where it rushes by whatever test article is placed therein.
The flow continues into another larger nozzle where it decelerates and passes through a heat-exchanger to cool it. The slowed air enters an exit plenum and then a low pressure chamber.
As air leaves the high pressure chamber, the pressure in the chamber decreases. Likewise, as air enters the low pressure chamber, the pressure in that chamber increases. Eventually, the pressure in the two chambers equalizes, the flow stops and the test is finished. The air or other gas such as helium or nitrogen in the low pressure chamber is drawn out via a vacuum pump or stream ejector and either exhausted into the atmosphere or recaptured for repeat use. Blowdown tunnels are useful for studying hypersonic aerodynamics and the heating environment around various shapes.
“We’re looking at how the vehicle flies and what type of aerodynamic heating environment it might encounter,” Berger says. Researchers using HYPULSE, which was donated to Purdue by Northrop Grumman in 2020 can do the same.
HYPULSE can also operate in a reflected shock mode. In this mode a shockwave is created by a high speed piston or as in HYPULSE, a detonation of driver gas bursting through a diaphragm into a downstream shock tube filled with a test gas. When the shockwave bursts into the shock tube it reflects off an end wall, forming a stationary region of test gas at high temperature and pressure. The resulting hot, high pressure gas is then expanded through a nozzle up to a target Mach number and flows across a test article.
In reflected shock mode HYPULSE can produce high temperature hypersonic flows but only for a very short duration of 1 to 10 milliseconds. This is used to study the aerothermodynamics of hypersonic flight and develop materials / surfaces that can cope. But for test time, HYPULSE can’t match the fourth type of hypersonic tunnel – the Arc Jet.
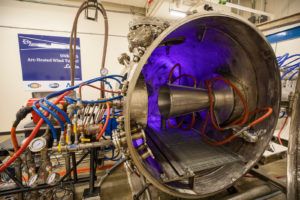
Arc-jet hypersonic tunnels flow super hot air, plasma, past test objects to simulate the friction at hypersonic speed which causes surfaces to heat up to more than 8,000K (about 15,000˚F). They can do this from seconds to minutes, so they are an optimal and critical tool for hypersonic materials engineering.
The University of Texas-Arlington completed an arc-jet hypersonic tunnel in 2019. Its 3000 to 5000K gas stream and maximum run duration of 90 to 200 seconds means it is in high demand from researchers.
Less in demand but perhaps the most high fidelity of all hypersonic wind tunnels is the ballistic range. Ballistic ranges are used to study aero-thermodynamics or impact dynamics. Instead of flowing hypersonic air past a test article, the object itself is propelled through the tunnel. Since the model essentially flies free, engineers don’t have to compensate for flow around a mounting apparatus, making data more real-world representative. The disadvantage lies in having to fire the test object via a detonative mixture or gun. Test objects may not hold a desired attitude throughout their flight, potentially wobbling down the tunnel.
NASA’s Ames Research Center and the Army’s Aberdeen Proving Ground operate ballistic range tunnels. Because objects move through them at such high speed, measuring variables such as flow characteristics, separation, temperature or boundary layers is difficult. Photographing the test object through a small window as it passes by is a feat of timing and the opportunity to place sensors on the article itself is limited.
In general, hypersonic tunnel and model instrumentation can be discrete, for example thermocouples or global, such as surface paints or flow field measurements using lasers. Instrumentation helps engineers understand the tunnel conditions and the conditions impact on the model being tested. This might include speed, density, pressure, heating.
“Lately you see a lot more facilities going to optical testing,” Berger explains. Temperature sensitive paint, infrared sensors, or two-color phosphor thermography, help engineers see where trouble points on a surface could develop.
Actual flight testing is obviously the most real-world hypersonic crucible but it’s expensive, hard to image and generally employs a small number of on-vehicle sensors. Data collection is mostly limited by insufficient downlink bandwidths. Furthermore, test vehicles are often not recovered. “A lot of them just go splash in the ocean,” Jewell says.
Hypersonics is simply hard. As no one ground facility can realistically simulate or measure all the parameters of Mach 5-plus flight, the West needs as many different types of hypersonic tunnels as it can get. That means making large investments.
The University of Notre Dame is in the process of erecting America’s largest Mach 6 quiet tunnel with a test section 24in (60cm) in diameter. Notre Dame puts the price tag at US$4.7 million. According to Berger you’d likely need a large amount of real estate to put it on. Support equipment for the hypersonic tunnels is “really loud.” And, she adds, the air coming out of another hypersonic tunnel at NASA Langley – the 8ft High Temperature Tunnel – during a run is “very loud!”