by Jack Roper
The sudden silence of the skies during lockdown has underlined the usual prevalence of aircraft noise. For years, noise pollution has steadily mounted as air traffic has escalated. Drones and air taxis aspire to fill the heavens, but their insectile whirr seems an insuperable barrier to acceptance. In Europe alone, exposure to road traffic noise is believed to cause 10,000 premature deaths each year. As aircraft propellers convert rotational speed into linear thrust, they create acoustic fields which we perceive as noise. This is both a pressing problem and an inescapable necessity of flight.
“Aerodynamic forces depend on pressure-changes,” says Czech Republic-based propeller manufacturer Woodcomp Propellers R&D Manager, Vilém Pompe. “But every lifting-surface which generates an aerodynamic force also becomes a source of noise. If no noise is radiated, no aerodynamic forces exist and no airplane or rotorcraft can fly.” If propellers are our only choice, then their noise can only be mitigated.
One way to mitigate propeller noise is to reduce the frequency of sound they emit. “The lower frequency of a Harley-Davidson makes it less annoying than a dentist’s drill,” Pompe says. A propeller developed for EU research project ARGOS (Aerospace propeller useful for diesel engines with extreme excitation of vibrations) last year produces a relatively tolerable noise-signature because of the low rotational speed of the diesel engine which drives it. Blade configurations can be tailored for acoustic performance, but incremental reductions depend on highly accurate wind-tunnel data and cutting-edge digital optimization. UK-based company Dowty Propellers, which can trace its history back to 1937 and produced propellers for the Spitfire WW2 fighter, is working to refine the technology.
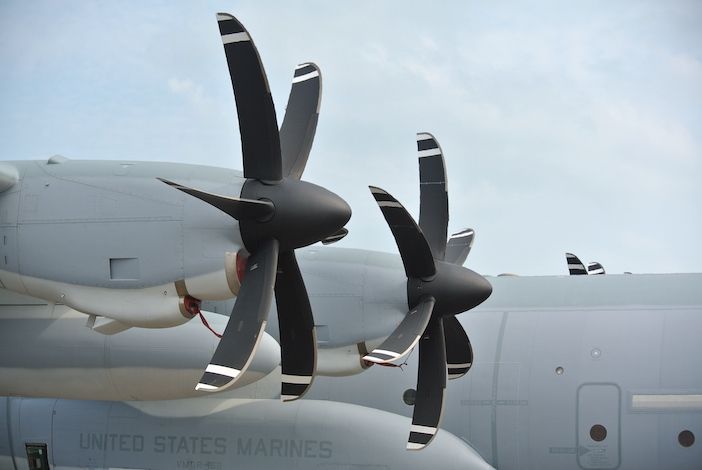
(Image: Dowty)
Blade shape
Today as part of GE Aviation Systems, Dowty produces propeller systems for the Lockheed Martin Super Hercules and De Havilland Dash 8. “Innovation out of necessity remains a trait of the business,” says Dowty’s technical director, Jonathan Chestney. “Regional aircraft require the lowest possible noise signature, both near-field within the fuselage and far-field, in terms of those living close to airfields.” As Dowty worked with progressively larger aircraft generating greater thrust, it evolved means to regulate propeller noise.
Dowty brought the world’s first all-composite blades into service in the 1980s and has made over 23,000 propellers from composites. “Composites afford us flexibility to tailor shapes to address acoustic aspects,” says Chestney. “All our propellers are variable-pitch and change blade-angle for different flight conditions, allowing us to tune the noise signature. Likewise, synchrophasing, whereby blades on twin- or four-prop aircraft turn in close synchronization, helps minimize fuselage noise-effects.” But Dowty’s quest for ever-quieter propellers drives continued aeroacoustic research.
UK government-funded research program Impacta began in 2012. As part of the project, wind-tunnel tests measured propeller efficiency and acoustics while the Royal Netherlands Aerospace Centre (NLR) ran tests examining how propeller noise translated into the cabin. The resulting data on transfer-function enables Dowty to simulate cabin-noise from different propellers, play it to students in a pod at Liverpool University and test their responses. “Impacta went from theory, through testing, to project how sitting in those noise profiles actually felt,” says Chestney.
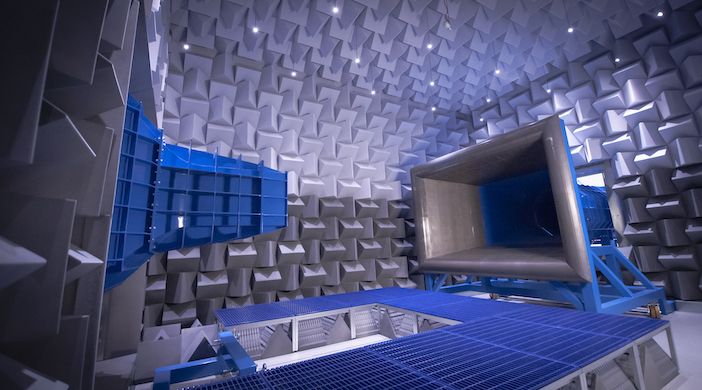
“We looked at changing relative blade positions, radially or axially, to adjust fundamental frequencies and create a more benign sound. But we wanted to understand how to feed that learning back into our prediction tools to inform future designs.”
Increasingly, Dowty uses computational fluid dynamics (CFD) to model the pressures involved in lift, drag and propeller efficiency. “The specific frequency of pressure-waves hitting the fuselage determines noise in the cabin,” says Chestney. “We’re developing our tools to account for noise-properties and enhance our predictive capabilities.”
Digital Propulsion, another government-funded project which commenced in 2017, will evaluate propeller technology across the whole lifecycle and develop the digital thread running from customer requirements, via design and testing to in-service data-collection and how that feeds back into digital tools.
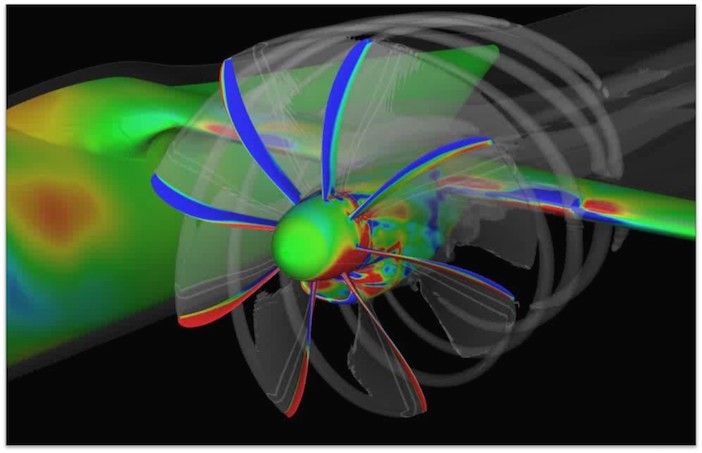
The role of tunnels
Dowty is also partnering on a research project to improve acoustic data quality with NLR and German-Dutch Wind Tunnels (DNW) called TASTE (Techniques for Acoustic Measurements in High-Speed Test Environments). Wind tunnels, long central to aerodynamics research, are indispensable to aeroacoustic studies that seek marginal improvements by testing different configurations in identical conditions. “Wind tunnel tests provide control and consistency,” says Dowty’s performance team leader, Ed Jinks. “They are the gold standard for quantifying designs. Numerical models will always need validation, because we need the confidence to apply them, both for ourselves and our customers.” But wind tunnels are enclosed and create reflected sound, which is difficult to disentangle from the true signal from a propeller.
“One microphone only measures one signal,” explains NLR R&D engineer, Evelien van Bokhorst. “It cannot say which part of the signal comes from the propeller, wind-tunnel noise or the interaction of propeller and inlet.” Current measurement techniques obviate this problem by using acoustic arrays. “We use 82 microphones, arranged in a certain distribution,” van Bokhorst says. “A soundwave from one source arrives at each microphone at a different time. From these phase-delays, we can construct where the sound comes from.” This technique’s dizzying precision relies on a DNW-designed data-acquisition system synchronizing measurements at 100kHz frequency.
Beamforming is a mathematical method originally developed to measure the spaces between stars. Acoustic beamforming algorithms cross-correlate microphone array results into sound-maps and isolate the propeller signal. But there are shortfalls involved. “We assume the source is a monopole: a single point-source,” says van Bokhorst. “But a propeller is not a monopole.” TASTE aims to produce more accurate and nuanced data by improving current beamforming algorithms. “We’re developing a steering function which models the sound-source more realistically, accounting for operating conditions like propeller rpm and number of blades.”
“Preliminary studies with advanced beamforming have enabled us to target specific noise-sources and areas of the blade,” Jinks reveals. “It will mean we can target our designs to improve upon those noise-sources.”
New measurement techniques
In addition to improved beamforming, a second part of the TASTE project is developing a way to capture acoustic signatures in wind tunnels using particle image velocimetry (PIV).
“PIV is a non-intrusive way to measure whole velocity-fields,” explains van Bokhorst. “Its components are a laser, cameras, and particles in the flow.” Pioneered at NLR, the system uses helium-filled soap bubbles, capable of seeding a wide area and following an air-flow exactly, without inertia distorting results. “We take two pictures of particles in the laser field, a short time apart,” she continues. “From the displacement of particles, we can calculate the velocity-field. By subtracting the momentum leaving the field from the momentum entering it, we calculate the force on the propeller, or blade-loading. Finally, we put the blade-loading into simulation software to infer the acoustic pressure-field.”
“Observing those bubbles should help us understand pressure variations at source-points around our propellers, which now we have to infer,” says Chestney. “TASTE will also enable us to extract real information from data more quickly in post-processing and use that to feed our analytical capability. Ultimately, that means we are confident in solutions prior to costly flight-tests and can exploit successful technologies in service.”
TASTE is testing its methods in NLR’s new aeroacoustic wind tunnel before progressing to test propeller configurations in DNW’s industrial low-speed wind tunnel. “Anything providing better performance at lower rotational speed is valuable, because low speed creates inherently lower noise-signatures,” says Chestney. “By adjusting blade-spacing, we might spread acoustic output across a broader bandwidth. As we begin to understand where different elements of acoustic output develop on the propeller, we could forgo performance in one part of the blade but recover it elsewhere, in order to reduce noise overall or avoid certain frequencies.”
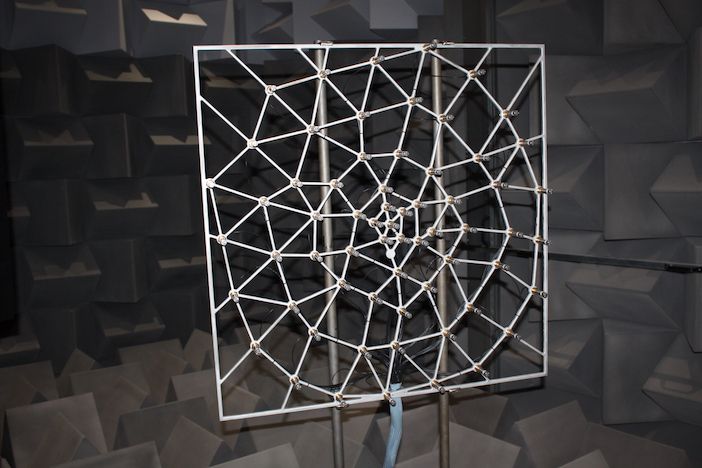
Vilém Pompe is more phlegmatic. “The means of reducing propeller noise are well-known,” he says. “Reduction of rotational speed and, in turn, the blade-tip Mach number. Increasing the number of blades to distribute pressure across the disk. Using thin and narrow blades.
“But we must pay for such improvements with reduced efficiency and increased weight. The most efficient propeller is a one-blade propeller, but from a noise perspective, that’s the very worst solution.”
But at Dowty, multi-disciplinary optimization (MDO) is promising a new way to weigh and resolve such complex trade-offs. “Based on customer requirements, we use an automated design process to determine the optimal blade-shape,” says Chestney.
“As we advance our analytical tools, we’re beginning to vary the distribution of blade-loading running from root to tip.
“Previously, we would create an aerodynamic design, then try to understand its acoustic properties and whether it was cost-effective or manufacturable. Now, that gets tied together in a single optimization process, giving us the best of all worlds in overall propeller-system performance.”
“Further breakthroughs require systematic change,” Pompe says. “Per partes optimization has reached
its limits and the only way to reduce noise is by optimizing whole aircraft – the airframe, engine and propulsor together.”
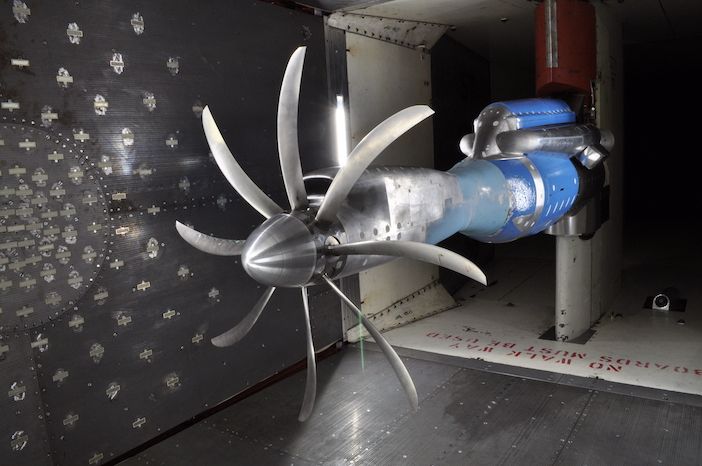
Chestney agrees. “It’s hard to make a difference by testing propellers in isolated free-air environments. We need to understand flows caused by the airframe,” he says. “The nature of aircraft may change, but integration only becomes more important in providing optimal propulsion for the aircraft of tomorrow.”
By doing so, Chestney hopes Dowty can return to its beginnings and recapture the happy splendor of its early work. “The Spitfire is one of the few aircraft people actually love to hear in the sky,’ he says. “If I had a goal in life, it would be for people to enjoy hearing aircraft again, instead of considering them noise pollution.”
ARGOS Project tests propellers with lower rotational speeds
Diesel compression-ignition engines have several advantages over the piston engines used today in most small aircraft. They use jet fuel, are less volatile and more widely available than Avgas for piston engines. Their higher compression-ratio makes them efficient enough to extend an aircraft’s operating radius by 25%, but also increases cyclic loading on the propeller. Since their rotational speed is lower, achieving the same power requires additional torque, increasing torsional vibration.
New propellers equal to such rigors are therefore a precondition to diesel adoption. Developing them was the focus of ARGOS (Aerospace propeller useful for diesel engines with extreme excitation of vibrations, a 40-month EU research project which concluded in 2019. Argos involved French engine-maker Safran and three Czech entities: Woodcomp Propellers, control-unit supplier Jihostroj and the Czech Aerospace Research Institute. It created a diesel-compatible wooden propeller with protective composite layer.
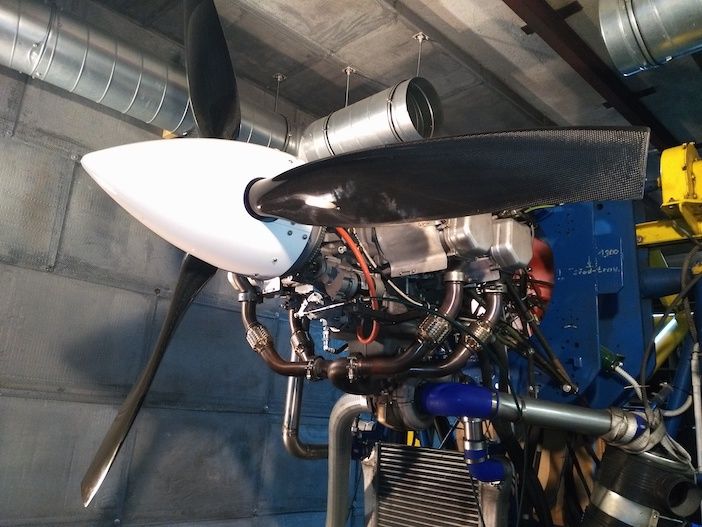
“We knew the propeller needed a relatively low polar moment of inertia to reduce its response to cylinder firing-effects, says Woodcomp’s Vilém Pompe, ARGOS Project co-ordinator. “We knew wood has excellent damping ability, doesn’t suffer fatigue and is sufficiently resistant to foreign-body impacts when equipped with composite skin.”
“We evaluated different composite skin reinforcement materials,” Pompe continues. “Torque is transferred through the propeller flange into the structure of the hub. The reaction to this is the aerodynamic force generated by the blades. These two effects intersect in the blade retention. Here we included retention bearings to absorb vibrational energy.”
The ARGOS propeller adopted a simple design with minimal stress-concentrators such as notches, which cause cracks and deformation when structures are loaded with forces. A modified prototype now in production will undergo certification and flight-tests could soon beckon. ARGOS collected data from various propellers with both petrol and diesel engines. This could prove invaluable to future innovation in an area where no previous data existed.
“We proved a structural solution to be possible without any additional damping device,” Pompe concludes. “We proved that aerodynamic design must respect diesel’s lower rotational speed to achieve the best propeller efficiency. With our experience from ARGOS, commercialization is a logical step.”
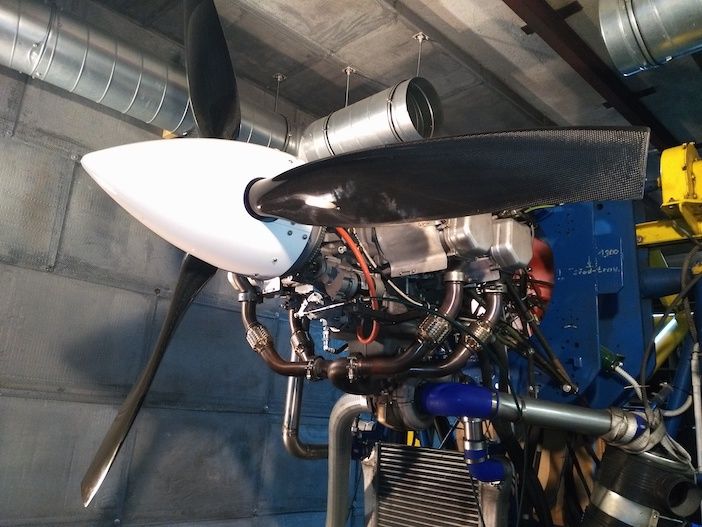
New materials offer sound advantages
Aluminum matrix composites (AMCs) are a class of next-generation materials with potential for propeller noise-reduction. AMCs consist of aluminum, reinforced with a secondary high-performance material. Lighter than traditional metals but with comparable structural integrity, their properties can
be tailored by particle or by continuous
fiber reinforcement.
“AMCs could preferentially change the noise, vibration and harshness characteristics of structures,’ says Gemma Christian, technical director of AMC supplier, Alvant. “Reduced propeller diameters equate to lower tip-speeds and therefore less noise. The lightness, strength, stiffness of AMCs could reduce manufacturers to add more blades to reduce that diameter.”
Commercial aircraft typically consist of 50% composites, 20% aluminum, 15% titanium and 10% steel. Besides lightweighting for better efficiency and reduced fuel burn, aerospace aspires to reduce reliance on titanium, which is costly, hard to machine and harmful to mine. AMCs promise an apt replacement.
“AMCs can make drivetrain-related components lighter and increase an aircraft’s useful load,” says Christian. “They can eliminate inertia, reduce thermal expansion coefficients and provide excellent tolerance to damage and fatigue.”
Alvant will design, manufacture and test an AMC brake rod as part of Large Landing Gear of the Future, a UK£28 million project led by Safran Landing Systems. Its overall aim is to deliver a 30% weight-reduction on current state-of-the-art landing-gear systems.
“We already have high-caliber aerospace-sector partners and anticipate more industry players eyeing the appeal of AMCs in multi-propeller solutions,” says Christian. “Propellers are one of the many components which can benefit from the properties
they afford.”