Words by Paul Willis
The largest airframe ever dropped at the NASA Langley Research Center’s crash test facility had the latest camera technology installed on it to help collect data and provide insights about aircraft structures.
The 150ft drop, on to a target of packed earth, lasted four seconds. Those few seconds were the result of nearly a year of preparation, says Dr Justin Littell, NASA’s lead researcher for the crash test.
“We started planning in July 2018 and we did the test during June this year,” he says.
The 33,000 lbs Fokker is the largest airframe ever dropped at Langley’s Landing and Impact Research Facility, which is also known as the gantry. The 240ft high steel structure has a pivotal place in the history of aerospace testing.
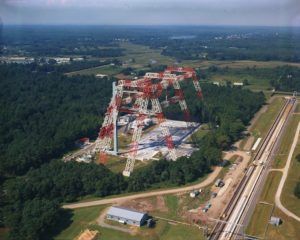
and Impact Research Gantry in 1969, during testing of the lunar module
Built in 1963, the gantry was originally called the Lunar Landing Research Facility and was where the Apollo astronauts trained to land on the moon. During the 1970s the field of aviation crash testing was pioneered here.
Research carried out at the Landing Research Facility, which is now a US national historic monument, contributed directly to the development of many aviation safety standards, says Littell.
“One of the major developments that came out of here was the dynamic seat test,” he says. “They carried out several full-scale crash tests here and with that data worked out the qualification standards for seats.”
The Fokker crash test was carried out by NASA and the Federal Aviation Authority (FAA). According to the FAA, the Langley facility has made a major contribution to aviation safety, particularly in helping aircraft makers understand how to manage the energy that is transferred to the occupants during a crash scenario.
While modern crash tests look similar to those done in the 1970s, the use of electronics and computer simulation has made the set up and data collection process vastly different.
Structural performance
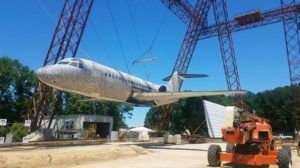
This summer’s crash worthiness test on the Fokker F-28 – a regional jet used on short to medium-haul flights – was conducted to advance research on the structural performance of this style and size of aircraft.
The third test in a series, the tests preceding it involved vertical drops of F-28 fuselage sections. The full aircraft test added the component of forward velocity to the vertical drop.
It did this by suspending the aircraft from cables set up in a pendulum configuration, so that plane’s trajectory during the drop was both horizontal and vertical. Planning for a complex test like this begins by asking yourself a series of questions, says Littell.
“We ask: What kind of impact conditions do we want? What kind of instrumentation do we want? What is technically feasible within the constraints of the facility? From these questions we develop all the different areas of the test.”
Instrumentation used in the test either monitored the aircraft or monitored the anthropomorphic test devices, the crash test dummies, on board.
Life of a dummy
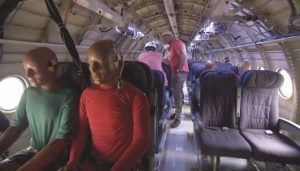
Littell’s team used a number of crash test dummies ranging in body types, from infants to obese male adults. The dummies were placed throughout the cabin. Special attention was put on the positioning of the dummies conforming to the 50th percentile, because this is the body type that the FAA uses in
its regulations.
The dummies were fitted with accelerometers in the head, chest and pelvis and load cells in the spinal column and neck. The load cells measure force, says Littell, “indicating compression in the spinal column and whiplash in the neck.”
As well as the sensors inside them, the dummies were also monitored using cameras mounted inside the cabin. The cameras allow researchers to correlate measurements from the body-mounted sensors with movements the dummies make.
“For example, the camera can show you that a dummy sunk in its seat and that’s why there’s a high load measurement on the load cell,” says Littell. “It is able to give us a complete picture of what the dummies experience on board.”
For the Fokker test, high-speed ruggedized cameras were trained on all the dummies except for the pilot. The cameras, which are designed to withstand high g-forces, were mounted to the cabin’s overhead racks using a gimbal system.
Littell’s team also used several high-definition 4K cameras to monitor both the dummies and deformation to the cabin that occurred during the impact. The aircraft was also fitted with accelerometers throughout the airframe – in the floor, in seat bases and overhead bins, and in the engine, tail and nose.
Such a high volume of instrumentation produces a lot of data. In total, the data acquisition system for the crash test used 300 channels. Outside of the aircraft, a further set of ground-based cameras were used to monitor the fuselage using a technique known as digital image correlation (DIC).
Seeing dots
The DIC process involves painting the exterior of the fuselage with black dots. Littell says, “We then run a calibration procedure on the cameras, which allows them to track the movements of these dots throughout the crash,” he says. “By measuring how the dots move relative to each other, we can calculate fuselage strain and deformation.”
Before DIC, displacement was measured by fitting strain gauges to the fuselage, an expensive and labour-intensive process, says Gerardo Olivares, director and senior research scientist at the US National Institute for Aviation Research (NIAR) in Wichita State University, USA. But DIC – helped by ultra-high-speed cameras, some of which can take up to half a million frames per second – allows for far greater definition, giving researchers a much clearer picture of “how stress and strain waves travel through the structure during a crash”, says Olivares.
To help clarify this picture software is used to analyze and display the level of displacement in color-coded graphics.
The NIAR provides certification testing for aircraft OEMs, many of which have a manufacturing presence in Wichita. The Institute is set to move to a new facility on the university campus early in 2020.
Virtual testing
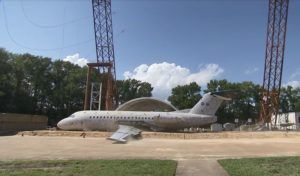
While the new facility will continue to carry out physical crash tests, Olivares says that the NIAR is moving increasingly towards virtual testing using computer simulations. “Once we can convince the authorities that the models are predictable, the idea is to replace full-scale physical testing,” he says.
He predicts that it will take around 15 years to spread the simulation models currently being used in their labs throughout the aviation industry. Although, as Olivares notes, testing with simulation models is already happening. “When they started building the Boeing 787 and Airbus A350 out of composite materials, the FAA was worried that they wouldn’t have the same safety standards as metallic airframes. So, they tried to get the two OEMs to carry out full-scale drop tests, but they refused,” he says.
“In the end they came to an agreement that they could drop test a section of the aircraft. If they were able to prove that simulations could accurately predict the damage to the aircraft section, then they could use simulations for full-scale testing.”
Both Boeing and Airbus were able to show simulations can accurately predict damage sustained during a crash. Furthermore, crash test simulations can also give engineers a level of detail impossible with physical tests, says Olivares.
“We’re working on developing human body models so we can better understand the injury mechanics,” he says. “Because with the level of complexity contained in some of these mathematical models, we can look at the crash impact right down to the level of organ tissue and bones.”
Another major advantage virtual testing offers is the possibility for near-infinite variability in the parameters of the test, says Olivares. Unlike with physical tests, planes crash-tested in simulations can be dropped onto any type of terrain and in any type of weather condition. “Simulation is going to bring us to a more real world understanding of the requirements because right now with the physical testing we’re working in a very constrained way,” he says.
The structural analysis techniques that inform the models were first developed for the automotive sector. Olivares says that while aerospace is generally conservative when it comes to changes to flight safety – a fact that he attributes to the heightened risk of fatalities associated with plane crashes – there is a growing understanding within aviation that the future of flight testing is virtual.
Tacit recognition of this can be found in Advisory Circular 20-146A, a document that the FAA put out in 2018 which offers guidance for certifying seats using computer modelling.
“The circular allows OEMs who are certifying aircraft to use simulations to identify worst case scenarios and then run physical tests on those worst cases. Whereas in the past they were required to run tests for multiple conditions,” says Olivares.