Words by Frank Millard
The requirements for the radiation testing of electronics and other equipment on space vehicles is changing as the space sector expands.
The exposure of spacecraft and their components to solar and cosmic radiation has posed considerable and complex problems as space exploration has progressed and matured. However, the technology to turn outer space into a safer environment for humans and machines is continually developing.
Space ionizing radiation originates from the Sun in the form of solar flares and coronal mass ejections, the two Van Allen belts, as well as cosmic galactic rays.
Spacecraft include structural materials and EEE (Electronic, Electrical and Electromechanical) components that can be affected by ionizing radiation. “Some parts are directly exposed to the external radiation environment. Some are located inside the spacecraft and are shielded by the external wall of the spacecraft to some extent.
The Radiation Hardness Assurance and Component Analysis Section (TEC-QEC) at the European Space Agency (ESA) supports the RHA and component analysis of all EASA projects.
Anastasia Pesce, head of the TEC-QEC, which is based in Holland says, “Some particles are so highly energetic that they will pass through several centimeters or more of aluminum shielding and directly pass through or accumulate in material and EEE components, which can lead to instant or progressive degradation of performance.”
There are three mechanisms of degradation on EEE components from ionizing radiation: total ionizing dose (TID), displacement damage (DD) and single event effects (SEEs).
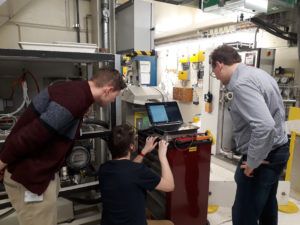
The effects of SEE range from being a nuisance to non-critical equipment to destroying critical hardware. The scope of work to make electronics more resilient to radiation is increasing for forthcoming missions to the Moon and beyond, because the environment is harsher in space outside Earth’s magnetosphere.
Pesce says, “Contrary to TID and DD, with SEEs a single ionized particle is capable of depositing enough charge in the device that can alter its operation temporarily or permanently. It can occur from the first second in-orbit and throughout the mission duration.
“Total ionizing dose and displacement damage are cumulative effects. TID generates from electron-hole pairs generated in the semiconductor oxides, while for DD the cause is due to energetic particles causing displacement of atoms in the component’s material lattice.”
Biological effects
The longer a component is exposed to radiation the more it degrades until failure. Mitigate measures are put in place by engineers, unless a component is able to function correctly for the entire duration of the mission.
Radiation shielding requirements thus depend upon the mission environment and duration. Spacecraft for human space flight also present another set of considerations. NASA has been concerned about the negative effects of radiation on spacecraft and astronauts for many years. Razvan Gaza, ionizing radiation lead, Lockheed Martin says, “The biological effects of ionizing radiation exposure constitute the limiting factor in terms of mission duration. It is unfeasible to deploy radiation mitigation strategies used on satellites for human space vehicles.
“The concept of a safe mode – shutting down non-essential functions while troubleshooting an SEE – is not applicable for environmental control and life support on a human spacecraft while the crew are onboard. Multiple redundancy, hazards- and operational controls are imperatives for NASA to ensure the safety of the astronaut crew during missions.”
Radiation sources
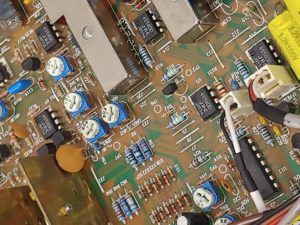
The testing of the effects of radiation and shielding can use several different approaches, depending on the type of effects under consideration. Facilities either use radioactive sources such as Cobalt 60, which emit gamma rays, or particle beams from particle accelerators which can be used to generate electrons, protons and heavy ions for use in testing.
Professor Nigel Bannister, senior lecturer from the School of Physics and Astronomy at the University of Leicester says, “If we are interested in how a material or an electronic component changes its properties over time because of ionizing radiation, then radioactive sources and beams of electrons and protons will be used.
“In the case of understanding how microprocessors are affected, then heavy ion beams and proton beams are used.”
Bannister’s work is currently focused on the ESA’s JUICE (JUpiter ICy moons Explorer), a probe which is planned for launch in 2022 and is scheduled to arrive at Jupiter in 2029. JUICE will spend at least three years making detailed observations of the giant gaseous
planet and three of its largest moons, Ganymede, Callisto and Europa.
“Jupiter’s strong magnetic field traps particles and the rotation of the planet accelerates these particles to high energies. This is what forms the radiation environment, which can damage and destroy electronics and materials,” says Bannister.
Testing of JUICE’s components consists of exposing the parts to a representative environment in an accelerated way. “To execute a TID test, the tested parts are exposed to photons emitted by the decay of a Cobalt 60 radioactive source. To test against DD, which is a particular challenge for optical and optoelectronic parts, the parts are exposed to high energy protons up to a certain fluence,” says Pesce.
For SEE testing, energetic ions or high energy protons are used. These deposit significant charge along their track when crossing semiconductor devices. This can induce SEEs ranging from temporary upsets to potentially destructive events such as latch-ups, which are similar to short circuits. Tests are performed at exposures vastly exceeding that expected in the mission to detect all possible failure modes. Testing is performed at EEE part level. The results of the test are then assessed in the context of the electronic circuit and up to system level to assess mission impacts.
Radiation testing is only one component of radiation hardening assurance (RHA). For mission critical electronic equipment, RHA is an integral part of the circuit and system design and engineering. RHA also plays a role in part selection, design for radiation mitigation, SEE rates and criticality analyses, and system integration of radiation effects.
Rad-hard or off the shelf?
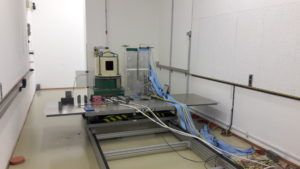
There is a trend for space vehicle developers to use COTS components instead of radiation hardened ones because of a reduction in the number of manufacturers producing radiation-hardened components. COTS components also often perform better in terms of mass, size, power and speed.
However, NASA scientists say COTS components can pose more risk for radiation effects as they are not subject to RHA. While radiation testing may characterize, it does not guarantee radiation suitability for a particular mission or environment. It can also be difficult to establish whether the quality of the manufacturing is consistent.
Pesce says, “There could be process variations, different wafers and masks used, different manufacturing plants. All these factors may change the way a component behaves when exposed to ionizing radiation. This is called lot-to-lot variations. Hence, the biggest issue is a lack of traceability of COTS.”
Johnson, research coordinator at the 88-Inch Cyclotron at the Lawrence Berkeley National Laboratory in California says, “With Cold War components depleted and no longer being made, there is a lot of interest in COTS parts for space vehicles. But they do not hold up as well in heavy ion testing. How manufacturers and space agencies are dealing with this is not always clear, but the need for more testing has clearly increased.”
Ringing the changes
As the requirements change the vulnerabilities and testing needs also change. According to Johnson electronic components are reducing in size, while complexity is increasing. This is increasing the demand for higher energies of particle beams to penetrate thicker silicon overlayers in components. New materials are also constantly emerging which require evaluation and assessment.
This means testing facilities are always looking to develop bigger, better beams. Johnson says, “We bring a new ion source online around every ten years which will use higher energy ion sources allowing us to increase overall beam energies. We are also looking at developing more precise particle beams, so that very small parts of components can be irradiated, rather than an entire circuit board assembly. This could mean far better diagnostic capability for testers.”
According to Gaza, the increasing complexity of EEE parts such as microprocessors makes characterization testing more difficult. He says, “Manufacturers are often reluctant to share detailed information about part functionality for radiation testing and testing black boxes is extremely challenging. Ultimately, a test aims to correlate results obtained in an accelerated environment with performance in flight. This is impossible without insight into the part functionality.”
This article was originally published in the March 2020 issue of Aerospace Testing International magazine. View in its original format here. Subscribe to the quarterly magazine for free here and to the weekly newsletter here.